Protein therapeutics have revolutionized the way we treat diseases, from cancer to autoimmune disorders. Market Data Forecast reports the market size exceeded $170 billion last year. Monoclonal antibodies are predicted to dominate 2023’s top-selling drugs by Evaluate Pharma.
Subcutaneous injections and intravenous infusions remain the main route of protein drug delivery.
However, protein therapeutics are prone to various forms of instability such as aggregation, degradation, and formation of particles. Particles can be a significant concern, as they may compromise the safety and efficacy of the injectables. According to the FDA, since 2017, 40% of recalls of injectable drugs were due to particulate contamination. Therefore, the ability to closely monitor particle formation is critical to developing control strategies and ensuring the quality, safety, and efficacy of injectable drugs.
What are particles?
Any injectable drug can contain diverse types of particles, which may come from external sources like biological materials, building materials, or personnel. Intrinsic particles are derived from within the drug product, and can come from processing, packaging materials, or product interactions.
Compared to non-biologic injectables, therapeutic proteins will contain proteinaceous aggregates, some of which are large enough to be quantified and characterized with nano- and microparticle counters. And excipient-related degradation products, like fatty acid particles, may also form due to drug-product interactions. They are considered inherent particles if measured and part of the clinical profile.
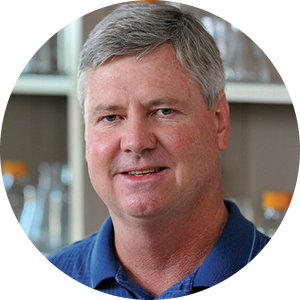
“Some of the main causes of [protein] particles are interfaces. And one of the biggest problems that we have in the industry is the damage caused on protein products by filling processes [where the protein formulation faces a lot of different interfaces],” said John Carpenter, Professor Emeritus at University of Colorado Anschutz Medical Campus, in his talk at the annual meeting of American Association of Pharmaceutical Scientists (AAPS).
Carpenter highlighted how some of the main causes of protein particle formation are the adsorption of protein molecules to interfaces, such as syringe walls or processing tubing. He emphasized that mechanical stress, agitation, compression, dilation, instability of the active pharmaceutical ingredient, and product-package interactions are all factors that can contribute to the formation of particles.
These inherent particles may pose a significant risk to patients, causing adverse immune reactions or decreasing the efficacy of the product. Therefore, it’s critical to accurately and reliably detect and measure protein particles. Their size range can be from a few nanometers up to hundreds of micrometers. This is especially important for particles not visible to the eye, called subvisible particles (SvPs), which require special analytical methods.
Analytical methods to detect and analyze subvisible particles are used throughout the whole drug development process, from formulation development to stability testing to release testing and post-market surveillance.
What universal method is capable of measuring particles across all size ranges throughout the entire drug development process?
There is no ultimate technology to provide data across the entire size range of particles.
One of the established and widely accepted methods for SvPs’ size analysis is light obscuration (LO), which meets regulatory requirements and is recognized as an official compendial method in many pharmacopeias. Although LO has historical data available, it has limitations in terms of protein particle detection. LO only provides count and size information, and it does not distinguish between protein particles and other particles. Moreover, LO may underestimate particles that are translucent, due to a low refractive index between the solution and particles. These are particles that are often formed due to interfacial stresses. Protein molecules form a film at interfaces, which can rupture for a variety of reasons, and release translucent particles that are hard to detect by LO.
To overcome these limitations, experts in the field use complementary methods, such as light microscopy, size-exclusion chromatography, nanoparticle tracking analysis (NTA), flow imaging microscopy (FIM) and other techniques to obtain a more comprehensive understanding of protein particle characteristics. Notable is that each technique measures particles using different properties, and inherent miscorrelations between different methods have to be accepted.
Rösch et al. noted that differences in outcome do not necessarily mean that one technique is more “right” or “wrong” than the other, but instead can be employed to gain a better insight into the particle content of a sample.
What novel solutions will we see in the future?
The outlook of protein particle characterization is bright, with several trends emerging in the field.
One trend that has been growing for more than a decade, is the use of multi-technique approaches to generate a fuller picture of particle characteristics. Many studies on this topic have concluded similarly that no single analytical technique can provide a complete understanding of particle properties and behavior. Therefore, efforts should be made to harmonize choices of methods across the industry to improve the comparability of results.
Machine learning algorithms are also being used more frequently to support identifying patterns in particle morphologies that may be difficult for humans to detect, leading to more informative results. We also see the integration and combination of techniques in a single instrument, such as directly combined systems of LO and FIM by FlowCam or the integration of Raman spectroscopy with imaging technologies. These types of instruments might further enhance protein particle characterization. Additionally, the development of new analytical methods like tunable resistive pulse sensing, reported by Wu et al., will continue to contribute to the field.
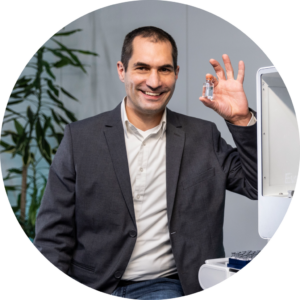
Although there are still limitations in the current techniques, such as those of LO to detect protein aggregates, the start-up company Bionter has focused on optimizing LO. The company has developed a non-destructive and automated way to leverage enough samples for comprehensive complementary analysis to allow multi-technique approaches. A further optimization of LO could be to rethink the light source to increase the sensitivity to protein aggregates, as Tobias Werk, founder and CEO of Bionter, outlined in his recent talk at AAPS.
Finally, with the increasing development of new protein drugs and other biologics (e.g. cell and gene therapies and lipid nanoparticle-based vaccines), there will be a need for faster and more efficient methods of particle analysis that can be implemented in real-time during the manufacturing process. This will require the development of in-line and online monitoring techniques that can detect particle formation and growth in real time throughout the production process.
Overall, the outlook of protein particle characterization is promising, with ongoing efforts to innovate and to ensure the safety and efficacy of protein therapeutics for the benefit of public health.
To learn more about Bionter AG and the company’s offering, please visit their website.
Author: Angelika Schrems
Images courtesy of Bionter AG and Shutterstock